Research Corner: Outdoor Lithium-Ion Energy Storage Systems
Lithium-ion (li-ion) batteries have contributed to a revolution in the electronics market since their introduction in 1991. They are lightweight, rechargeable, have high energy density and became much more economical in the past few years. These traits, along with higher demand on electrical grids and increasing renewable energy deployment, led to the adoption of li-ion batteries in grid-scale energy storage systems (ESS). Li-ion battery ESS feature large arrays of cells, which can total thousands of pounds per rack.
Li-ion batteries comprise an anode and cathode that are separated by a polymeric separator. The batteries generally are a safe technology, although abuse that leads to separator damage or cell overcharge can cause an internal short circuit, which results in self-heating. At a high enough temperature, exothermic reactions that occur within the cell contribute additional heat. The temperature increases rapidly in an uncontrolled process that’s referred to as thermal runaway, which causes the cell to thermally decompose into flammable gases, which might or might not ignite immediately. The heat can damage adjacent cells and cause cascading thermal runaway. This phenomenon was seen in highly publicized incidents of hoverboards and e-bikes burning or acting as an ignition source for larger fires.
The gases that are produced in a thermal runaway event are flammable and high in CO and CO₂ and might create explosion and asphyxiation hazards if they accumulate in a confined space. Because the number of individual cells that are in an ESS is manyfold greater than the number of cells that are in a hoverboard or e-bike, the potential incident scale also is much larger in an ESS.
The incident
UL’s Fire Safety Research Institute reviewed the April 19, 2019, explosion that occurred because of cascading thermal runaway within a 2.16 MWh li-ion battery ESS in Surprise, AZ. Four career Peoria, AZ, Fire-Medical Department hazmat team members received serious injuries from the explosion.
The 50 x 14.25 x 13-foot ESS structure housed 27 racks. Each rack held 14 battery modules. Each module had 28 individual li-ion cells (10,584 total cells).
Abnormal conditions in the ESS first were indicated when the smoke detection system signaled an alarm condition at approximately 4:55 p.m. An engineered suppression system discharged a total flooding clean agent suppressant 30 seconds after the alarm condition. The first firefighters (from the Surprise Fire-Medical Department) arrived on scene at 5:49 p.m.
The hazmat team arrived on scene at approximately 6:28 p.m. and noted low-lying white vapor clouds issuing from the structure and drifting through the desert. The team conducted multiple 360-degree size-ups around the ESS using multi-gas meters, colorimetric tubes and thermal imaging cameras. Concentrations of hydrogen cyanide (HCN) and carbon monoxide (CO) that were above the levels that are defined as immediately dangerous to life and health (IDLH) were measured throughout the vicinity of the ESS.
The hazmat leadership developed an incident action plan with input from senior fire officers and information that was provided by the owners, designers and maintainers of the ESS. The hazmat team approached the ESS at approximately 7:52 p.m. and found that the HCN and CO concentrations that were outside of the structure had decreased to ambient conditions. In following with the incident action plan, the team opened the door to assess the conditions inside of the ESS at approximately 8:01 p.m. A deflagration event was observed by the firefighters outside of the Hot Zone at approximately 8:04 p.m., which was three minutes after the door was opened.
On-site observations revealed that thermal runaways were confined to a single battery rack. However, it generated enough flammable gas to enable an explosion more than three hours after the initial alarm. The explosion deformed the ESS structure, caused the doors to become projectiles, and propelled one firefighter 73 feet and another firefighter 30 feet into the desert.
The experiments
In July 2020, UL conducted three research experiments in a 20-foot intermodal container that was built to represent an outdoor modular walk-in li-ion ESS, to advance the understanding of ESS fire and explosion hazards for the fire service, fire protection engineering and energy storage industries.
The three research experiments were planned and conducted with significant influence from what was learned in the review that was conducted of the Surprise incident. Specifically, the experiments were designed to document and demonstrate the fundamental fire and explosion hazards that develop when cascading thermal runaway occurs inside of a containerized li-ion ESS.
Each experiment was started by heating li-ion cells until thermal runaway began to cascade from cell to cell. Gas measurement instruments, such as those that might be present in an ESS installation and that are carried by the fire service, were incorporated to evaluate their measurement response for initial and ongoing incident size-up use. Deflagration venting was employed to enable safe demonstration of any observed deflagrations, indicating the potential explosion hazard that an unvented deflagration might cause. There were no interventions taken in the first experiment. In the second experiment, a total flooding clean agent system that was similar in design to the system that was in Surprise, was incorporated. In the third experiment, a water spray suppression system was employed to model an approach that was taken by some manufacturers to meet New York City ESS permitting requirements.
The tactical considerations that were determined through these experiments are:
- Thermal imaging cameras don’t enable evaluation of the number or location of ESS units in thermal runaway
- Thermal imaging cameras enable a limited ability to determine whether a suppression system has operated or is operating
- Thermal imaging cameras aren’t a viable tool for determining the nature of visible vapors (e.g., battery gas, steam, etc.)
- Responding firefighters should use portable gas meter measurements of CO and visual observations of characteristic low-lying white vapors to define a Hot Zone while wearing full structural PPE (Level D Ensemble) and full SCBA
- Fire service portable gas meters have limitations in a battery gas environment
- Ventilation of an ESS installation might result in a deflagration or rapid transition to flashover
- Using portable gas meters to evaluate interior conditions or the gases/vapors that leak from an ESS places firefighters in an explosion hazard area
- Commercial combustible gas and hydrogen detectors were effective for indicating thermal runaway off-gas but weren’t reliable for an ongoing gas hazard assessment; fire service portable gas meters typically use the same sensor technologies, which are subject to the same limitations
- The total flooding clean agent suppression system didn’t stop cascading thermal runaway or eliminate the explosion hazard
- The ceiling-based water spray suppression system limited cascading thermal runaway to a single rack but had limited effectiveness in suppressing the initiating rack
- The safest initial action at ESS installations with remote fire department connections is to supply the connection with water
Additional findings of the review and tactical considerations that were revealed are available in greater detail through the full-length report.
The Surprise, AZ, ESS Fire Factors
UL’s Fire Safety Research Institute identified the following factors that contributed to the incident and injuries that occurred on April 19, 2019, at an energy storage system (ESS) in Surprise, AZ:
- Core training curricula for firefighters and hazmat specialists don’t cover basic ESS hazards; extracurricular ESS-specific training doesn't comprehensively address ESS hazards
- There were no means for the hazmat team to monitor toxic gas concentrations, lower explosive limit or the conditions that were inside of the ESS from a physically secure location; additionally, the ESS communication system didn’t contact the fire department upon detection of an alarm condition, and the system failed early in the incident, which limited the amount of information that fire service personnel had about the ESS system
- The emergency response plan wasn’t provided to the responding fire service personnel prior to the incident, and the plan that was provided on scene didn’t provide adequate guidance for mitigating thermal runaway, fire and explosion hazards that were generated by the ESS
- The design of the ESS didn’t include deflagration venting or adequate mechanical ventilation to prevent accumulation of flammable gases above an explosive concentration; the total flooding clean agent suppression system prevented flaming during the early phase of the incident but didn’t arrest thermal runaway or provide explosion protection
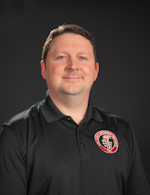
Adam Barowy
Adam Barowy is a lead research engineer at UL's Fire Safety Research Institute (FSRI). In nine years of commercial fire research prior to joining FRSI, he worked to develop UL standards and test methods and evaluated emerging fire hazards and new and innovative fire protection solutions. Barowy advanced UL’s capabilities for characterizing the thermal, fire and explosion hazards of energy storage technologies and led the technical development of UL 9540A and UL’s large-scale ESS fire testing program. Prior to UL, he worked at National Institute of Standards and Technology, where he conducted full-scale structure fire field experiments to enable improved firefighting tactics, reconstructed LODD/injury fire incidents and evaluated firefighting equipment. Barowy volunteered with the Amherst, MA, Fire Department while he pursued his bachelor's degree in mechanical engineering at the University of Massachusetts Amherst. He later graduated from Worcester Polytechnic Institute with a Master of Science in fire protection engineering.
Mark McKinnon
Mark McKinnon has a master's degree in fire protection engineering and a Ph.D. in mechanical engineering from the University of Maryland. He joined UL's Fire Safety Research Institute from the consulting world, where his work involved fire modeling, performance-based design, fire-related litigation, environmental regulation consulting, material flammability testing and experimental design.
Jack Regan
Jack Regan holds a bachelor's degree in civil engineering from the University of Illinois and a master's degree in fire protection engineering from the University of Maryland. He has worked on projects that examined occupant tenability and firefighter training.