International Exchange: Understanding Gas Cooling
One way to deal with this pesky smoke layer would be to ventilate the smoke and remove the potential danger out of the building. However, given the fact that most residential fires are ventilation-limited upon arrival, ventilation might cause more harm than good. Another solution would be to seize or diminish the production of the harmful gases by putting water on the fire as fast as possible. Applying a short exterior attack will greatly reduce the heat release rate and therefore also the heat that is being transferred into the smoke layer. One last method to reduce the risks is to prevent the ignition of the gaseous layer while advancing toward the seat of the fire. One technique that excels at this is gas cooling.
This article will focus on the European/Australian/Asian method of application and will be referred to as European gas cooling, as this is where it originated.1 There are certainly other methods, such as straight stream gas cooling or surface cooling, and it is not the intent of this article to advocate for one tactic or another; it is rather an attempt to explain how European gas cooling is performed and its underlying physics.
Gas cooling origin
Following the line-of-duty deaths (LODDs) of two Stockholm firefighters due to flashover in 1982, Swedish fire engineers Mats Rosander and Krister Giselsson proposed a new method of fire attack to prevent firefighters from being exposed to flashovers.2 Based on previous research of Layman and Herterich,1 they decided to further expand the principles of the indirect attack. This technique, originated in marine firefighting, requires a large amount of steam production by focusing the water application on the hot compartment’s surfaces in order to create a nonflammable atmosphere. Rosander and Giselsson, however, focused their research on cooling the smoke gases only, rather than displacing and diluting the atmosphere by mass steam production. This controlled manner of smoke cooling proved to change the landscape of European, Australian and Asian firefighting, as this resulted in their standard technique of advancement—that’s right, an advancement technique, not an extinguishing one.
Cooling basics
Gas cooling is performed by applying water into the smoke layer, attempting to touch the surfaces as little as possible. The main objective is to cool and dilute the overhead gases in order to prevent them from burning, but also to create a more favorable firefighting environment as firefighters advance toward the fire. It is important to reiterate that this is a protective measure to advance toward the fire compartment and should not be used as an extinguishing technique.
As the interior crew progresses to the fire compartment, they apply several bursts of water in a fog pattern (also known as pulsing), trying to cover as much volume of the fire gases as possible. Since the smoke generally flows in the direction of the firefighters as they approach the fire, it is important to try to cover the entire width of the room to prevent any uncooled gases to pass by them. It is also favorable to reach as far as possible since the cooled gases will come toward the nozzle operator. Therefore, the nozzle should be held at an angle of 45 degrees with the floor, as this will result in the greatest reach. A cone angle of 60 degrees is suggested, as this generally lets you cover the entire width of a room with two or three pulses. However, these angles are only guidelines and should be adapted accordingly to the room geometry. A smaller cone may be needed in a large space of a narrow hallway. The number of pulses should be adapted accordingly in order to cover the width of the room.
The gas flow moving away from the fire is also the biggest reason why it is important to advance after you’ve cooled the smoke. In small-scaled laboratory setups, fire gases typically reheat back to their initial temperature after roughly 5 seconds.3 If it is possible to close the door of entry, reheating will be slower, as the cooled smoke can no longer exit behind the crew and remain there as a buffer.
Because gas cooling is performed by pulsing controlled volumes of water, the flow rate is of lesser concern, as the nozzle operator can adapt the performance by opening the nozzle for a longer time. It is often asked how much water should be used. However, it is very difficult to give an exact answer. There are many variables, such as room geometry, smoke temperature, efficiency of the nozzle operator, etc. In Belgium, for example, firefighters are learning to flow for approximately 2 seconds every pulse for an average enclosure. They can shorten this in small hallways and reduce the nozzle cone accordingly or open it for a longer time when they notice that the short pulses have no effect. If no water droplets fall back, one can be assured that the room is very hot and a longer application can be more adequate.
Underlying physics
Cooling capacity
As water travels through hot smoke, the temperature difference between the two will induce a heat transfer from warm smoke to cold water to meet an equilibrium temperature. The water will rise in temperature by draining heat from the hot smoke. At 100 degrees C (212 degrees F), the water will evaporate. The evaporation process requires a huge amount of heat compared to the released heat from lowering the temperature of smoke by 1 degree C.
Table 1 presents how much smoke can be cooled for every liter (0.26 gallons) of water at 100 percent efficiency.4 Of course there is no such thing as 100 percent efficiency, but even at 50 percent, the results are very effective. If all water is vaporized and no equilibrium temperature is reached between the steam and smoke, the steam will continue to drain heat until they reach an equal temperature.
Because steam is introduced in the smoke layer, one of the misconceptions exists that the overall volume of this layer will increase. One liter of water turns into 1,700 liters (264 gallons) or 1.7 m3 (35.3 ft3) of steam at 100 degrees C. So, there is a fair amount of steam added to the mixture. However, cooled gases also contract and, in theory, the cooled smoke-steam-mixture has a smaller volume, even though an extra gas is introduced. Because the area of the room remains equal, this would imply that the smoke layer would rise in height. In practice, this contraction will only be local and will have a negligible effect on the total smoke volume of the complete enclosure. Nonetheless, the conditions will not worsen, which is contrary to what often is believed.
Large surface area
The method of application originated using a fog pattern. Using a small cone of approximately 30–60 degrees, a larger volume of smoke gases is subjected to water droplets compared to a straight stream. It is also favored to have small droplets. Smaller drops have a larger surface area in relation to their volume and so heat up and evaporate faster, consequently absorbing more heat.5
Dilution and thermal ballast
It can be argued that “smoke cooling” would be a better term to use than “gas cooling,” as what is being cooled is not only gas. Instead, smoke is a mixture of gases, liquids and particles. It could also be argued that a more correct term would be “smoke composition changing” or “gas changing,” as the smoke is not only cooled but also diluted and the composition is changed. However, the term gas cooling is widely used in the fire service community and will be further used in this article.6
As water evaporates by hot smoke, steam gets added to the complex gas mixture. Because water is non-combustible, it decreases the flammable range. Next to oxygen and fuel, the gas mixture in the smoke layer also needs energy to start combustion. Because steam is now also a part of this mixture, the provided heat from the fire will be distributed over steam, fuel, oxygen and inert gases. Consequently, more heat is required to bring the fuel-oxygen mixture to its flashpoint temperature (= lowest temperature at which a substance vaporizes into a gas, which can be ignited with the introduction of an external source of fire).
Operational benefits
Lowering the temperature of a flammable gas also results in a rise of its lower explosive limit. The flammable range of a gas is dependent on the mixture’s temperature. The lower its temperature, the smaller the flammable range and thus the higher the lower explosive limit (Figure 3).7 This implies that more energy should be produced by the fire before the smoke layer can ignite. Additionally, the added steam will react as a thermal ballast, which requires even more heat before the mixture reaches its flashpoint.
Lastly, because European gas cooling implies a controlled application of water, typically there is relatively little water used. The average residential fire does not require a large amount of water and neither does gas cooling.8 In Europe, it is common that engines have a small internal tank of water (+/- 250 gallons). The operational advantage of using only as much water as required is that tank water lasts longer. For many stations, this means that the second-arriving engine can establish a water supply. This way, the attack crew can be put in action as fast as possible while additional crewmembers simultaneously start search-and-rescue operations. Because every fire is different, it is up to the crew commander to decide if immediate water supply is required.
Misconceptions
Having a critical take on things is an important asset; however, there are several misconceptions on gas cooling to address.
As previously explained, gas cooling is not an extinguishing technique. There are several videos where firefighters can be seen standing before a door opening of an (acquired) house fire, applying short bursts of fog while there is a fully developed fire at the other side of the door. This is not an appropriate application of this technique. Gas cooling is a way to control your environment as you advance toward the fire room. Once you reach the fire room, the interior crew should transition to a direct or indirect attack to extinguish the fire.
Another thing that is often seen is stationary gas cooling. If a fire is compared to a disease, then fire gases can be associated with the symptoms. One may fight these symptoms posed by an illness, and the effects of the treatment will ultimately wear out unless it is treated at the source. Likewise, you may cool smoke as much and as long as possible from a stationary position, but you won’t extinguish the fire if you do not reach the base of it. On the contrary, cooling from a stationary position may have its place in training to show the effects of gas cooling.
Lastly, gas cooling should not be confused with the indirect attack. An indirect attack has the means to completely fill a room with steam by hitting as much hot surfaces as possible. Consequently, this adds a massive amount of thermal ballast, resulting in a decreasing fire. Gas cooling implies a coordinated water application aimed at cooling and diluting the gas layer without creating excess steam. This results in a controlled environment as the team moves toward the fire room.
Closing remarks
Gas cooling has a high learning curve and requires practice to result in the desired effects. However, the investment can pay off greatly by the added safety of the interior crew.
With the help of science, firefighting knowledge and technology are advancing at an exponential rate. At the same time, social media allows for a better distribution of knowledge and contribution to it resulting in an even higher pace. The UL studies on surface cooling, among other things, are such a valuable addition toward providing a safer environment for the interior crew. These studies are well looked at across the ocean and will definitely provide useful information for our tactics as well.
References
1. Grimwood, P. “Fog Attack: Firefighting Strategy and Tactics—an International View.” 1992. FMJ International Publications,
2. Grimwood, P, Hartin, E, McDonough, J, Raffel, S, “3D Fire Fighting Training, Techniques and Tactics.” 2005. Fire Protection Publications.
3. Van de veire, M. “Studies on the importance of firefighters’ gas cooling (report 5515).” 2016. Lund University: Department of Fire Safety Engineering.
4. Särdqvist, S. “Water and other extinguishing agents,” 1st ed. 2002. Swedish Rescue Services Agency.
5. Svensson, S. “The Operational Problem Of Fire Control.” 2002. Lund University.
6. Svensson, S, Van de veire, M. “Experimental study on gas cooling in firefighting operations.” 2018. Submitted to Fire Technology.
7. Lambert, K. “Flammability limits.” 2017. www.cfbt-be.com/nl/publicaties/artikelen.
8. Zevotek, R, Stakes K, Willi, J. “Impact of Fire Attack Utilizing Interior and Exterior Streams on Firefighter Safety and Occupant Survival: Full Scale Experiment. 2017. UL Firefighter Safety Research Institute, Columbia, MD.
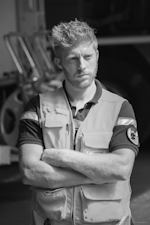
Matthias Van de Veire
Matthias Van de Veire has been a leading volunteer firefighter/EMT within his home town, Aalter, since 2007. In May 2017, he started his professional career as a battalion chief with the Brussels Fire Department in Belgium. Inspired by fire, science and teaching, he obtained a bachelor's degree in education and a master’s degree in fire safety engineering. Van de Veire emphasized his work on experimental studies, covering gas cooling tactics.