Research Corner: Lithium-Ion Battery Heat Release Rate and Explosion Hazards
Lithium-ion (li-ion) batteries are everywhere. They are in personal electronics, power tools, micro-mobility devices, electric vehicles (EVs) and energy storage systems (ESS). Researchers around the globe have been working to characterize the hazards that are generated from li-ion batteries during a thermal runaway. The hazards range from chemical and thermal exposures to explosions.
One of the common types of li-ion batteries that’s in use is the 18650 cell. These cells are cylindrical in shape and are 18 mm (0.71 inches) in diameter and 65 mm (2.56 inches) long. They can be based on different li-ion chemistries, such as lithium nickel cobalt aluminum oxides (NCA) and lithium iron phosphate (LFP). The NCA 18650 battery is used widely and has a nominal voltage of 3.7 volts, 3.5 ampere-hours (Ah) and 12.95 watt-hours (Wh).
A single 18650 battery cell can be found as the power source for personal electronics, such as a vape pen and a shaver. Three 18650 batteries might be used to power lights, speakers and toys. Six cells might be used to power a laptop computer. Ten 18650 cells commonly are used as the energy source for power tools, such as drills and saws. Battery packs that have dozens of cells are used to power micro-mobility devices, such as stand-up scooters. Larger battery packs that have as many as 100 cells are used to power lawn equipment, such as lawnmowers. Battery packs that have 136–150 cells can be found powering sit-on scooters.
HRR of NCA 18650 battery cells
Table 1 provides a range of peak heat release rates (HRR) for NCA 18650 cells that were overheated to cause a thermal runaway. The cells that were tested were at 100 percent state of charge.
Except for the single-cell version, the peak HRRs in Table 1 were measured for the Fire Safety Research Institute (FSRI) by UL Solutions, and the values are rounded up to the nearest 5 kW. As noted, there isn’t a linear relationship between the number of batteries and the peak HRR. This is a function of the propagation of thermal runaway within the battery pack arrangement. The thermal propagation is affected by many factors, including the arrangement and orientation of the cells, how many cells are involved in initiating thermal runaway and the type of enclosure.
Figure 1 shows the 10-cell battery pack arrangement during its peak HRR. Most of the cells are involved in off-gassing and burning at the time that the photograph was taken. Figure 2 shows the 152-cell configuration near its peak HRR; many of the cells hadn’t yet vented and burned.
Thermal runaway effluent gases
Although the HRR rate is one hazard from a li-ion battery thermal runaway that the fire service and the public must be aware of, as noted above, the potential for an explosion that’s generated by the thermal runaway effluent gases (TREG) is another.
In the HRR examples that are described above, the gases that vented out of the batteries during thermal runaway quickly ignited and burned off. As explained by Adam Barowy et al in “Explosion protection for prompt and delayed deflagrations in containerized lithium-ion battery energy storage systems” (Journal of Loss Prevention in the Process Industries, Volume 80, December 2022), the TREG from the NCA battery chemistry is composed mainly of hydrogen (33 percent), carbon monoxide (36 percent), carbon dioxide (22 percent) and methane (9 percent). Each 18650 cell can generate approximately 8 liters of TREG. If this TREG accumulates in an enclosed space, an explosion can occur.
Explosion hazard study
To examine li-ion battery explosion hazards, a residential garage was selected, given that such a premises is a location where li-ion batteries might be commonly stored, charged or used. The li-ion batteries could be in power tools, scooters, EVs or energy storage devices. (For a full description of these experiments and the resulting data, see doi.org/10.1016/j.fuel.2024.132818. To learn more about FSRI’s battery safety study, see fsri.org/research/impact-batteries-fire-dynamics.)
Experiments were performed at an outdoor explosives test site. The enclosure was designed to represent an attached residential two-car garage. (This research effort followed a U.S. Department of Energy project that demonstrated that releases of TREG from a residential ESS could create deflagration hazards. In that project, U.S. Census Bureau data was analyzed, and it was determined that the most common North American house that was built in the past 40 years typically was constructed as a tract house, which includes an attached two-car garage. This style of construction employs lightweight platform construction combined with wood-stud framing.)
The interior walls and ceiling of the test-site garage were finished with 0.625-inch-thick Type X gypsum wallboard. Wallboard seams were sealed with paper tape and joint compound.
Adjacent rooms were constructed on the rear and to one side of the garage to represent the structural reinforcement that normally is provided by the remainder of the house.
The structure was anchored to a concrete pad. A 16 x 7-foot steel overhead door was installed at the front of the garage, and a 30 x 80-inch steel-over-polystyrene core, 20-minute fire-rated interior door was installed in the center of the opposite wall.
Based on the composition and amount of TREG that’s generated by an NCA 18650 cell, bags were filled with simulated TREG, with volumes that spanned amounts that potentially are generated by a battery pack for a handheld power tool (124 liters of gas) through to a battery pack for a large piece of lawn equipment or a sit-on scooter (1,238 liters of gas). Once the bags were filled with the appropriate mixture and amount of hydrogen, carbon monoxide, carbon dioxide and methene, the bag was ignited remotely, which set off the explosion.
Observed damage
Throughout the series of experiment, cosmetic and structural damage to the structure occurred. The damage was repaired between experiments. The garage door served as a pressure relief panel. Figure 3 shows the garage door being blown off of the garage test prop by the gas that could be generated by a 100-cell (or larger) battery pack.
A range of damage was observed during testing. Even when damage wasn’t structurally critical, it compromised the fire resistance of the wall and ceiling assemblies that were between the garage and the attached house. In the event of a prolonged fire post-deflagration, the designed fire resistance of the garage space no longer would prevent spread into the residential space as intended.
Cracking of the sealed joints between drywall panels was observed in almost all experiments. In the case of the explosion that represented the 100-cell battery pack, in addition to the overhead garage door being blown off, some of the wall studs were cracked, and the interior door and latch were damaged.
The range of overpressures for gas volumes representing a 10-cell–100-cell battery pack was approximately 0.25 psi (1.7 kPa)–0.9 psi (6.3 kPa).
Another size-up concern
Firefighters have experienced li-ion battery explosions in apartments, garages, battery ESS and EVs. The research demonstrates the amount of energy and overpressure that can be produced during such an event. Therefore, large li-ion batteries are yet another fuel package for firefighters to consider as part of their ongoing size-up. Furthermore, firefighters should reconsider standing in front of a garage door that might have hot li-ion batteries on the other side (see “CO Firefighters Narrowly Escape Blast When Water Hits Battery”).
Interested in how to prevent li-ion battery thermal runaways in your home and in your community? Visit the FSRI Take C.H.A.R.G.E project resource page for first responders.
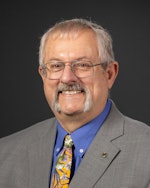
Dan Madrzykowski
Dan Madrzykowski is the senior director of research for the Fire Safety Research Institute (FSRI), which is part of the UL Research Institutes. He has a doctorate in fire engineering from the University of Canterbury and a master’s degree in fire protection engineering from the University of Maryland. Madrzykowski has more than 35 years of experience working to improve fire safety by conducting research and development in areas of fire dynamics, fire test methods, fire control and fire investigation. He is a member of the NFPA and serves on the committees for Fire Service Training, Structural Fire Fighting and Fire Investigation. Madrzykowski is a member of the International Fire Service Training Association's Executive Board and has collaborated with the International Association of Arson Investigators, International Association of Fire Chiefs, International Society of Fire Service Instructors and the National Fire Academy in the development of training programs. He is a Fellow with the Society of Fire Protection Engineers.
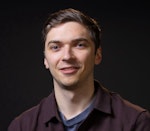
Nathaniel Sauer
Nathaniel Sauer is a postdoctoral researcher for the Fire Safety Research Institute (FSRI), which is part of the UL Research Institutes. He holds a bachelor’s degree in mechanical engineering and PhD in fire protection engineering from Worcester Polytechnic Institute. At FSRI, Sauer is in the nonstructure fires research group and has worked on full-scale burn and suppression tests of electric vehicles, explosion hazard analysis from battery thermal runaway gas, e-mobility device failure in commuter railcars and stranded energy dissipation of fire damaged batteries.